Can dopamine dysregulation cause eating disorders?
Eating behaviour is a type of motivated behaviour directed towards the goal of acquiring energy, and varies over different contexts (Higgs et al., 2014). Disruption to eating can result in eating disorders (Weiss, 1995; Higgs et al., 2014). Eating disorders (EDs) are characterised by clinically impaired psychosocial and physical functioning (Treasure et al., 2020), altered eating, and disturbed weight-control practices (American Psychological Association [APA], 2013). EDs include bulimia nervosa (AN) and anorexia nervosa (AN). BN involves repeated binge-eating followed by compensatory diuretic/laxative use and/or self-induced vomiting (APA, 2013). Whereas AN is characterised by low body weight for developmental stage, and persistent food restriction which causes severe health complications (Brumberg, 1988; Higgs et al., 2014). Whilst the true prevalence of EDs is unknown (Qian et al., 2021) around 20 million women and 10 million men in the USA are estimated to have an ED during their lifetime (National Eating Disorders Association, 2022). Given the estimated prevalence of EDs and the high associated mortality rate (Arcelus et al., 2011), research has proposed causal mechanisms for EDs. As socio-cultural explanations cannot explain why only a small proportion of individuals exposed to socio-cultural risk factors develop EDs (Nunn et al., 2012), alternative monoamine explanations have been proposed. This essay discusses evidence for dopamine dysregulation in AN and BN, followed by its involvement in the transition between EDs. Overall, AN and BN are characterised by different patterns of dopamine dysregulation, however causality cannot be confirmed.
In typical eating behaviour dopamine is involved in reward. Reward is comprised of ‘wanting’ defined as instinctive desire, and ‘liking’ defined as hedonic experience (Robinson et al., 2015). Dopamine underpins food ‘wanting’, whereas opioid signalling underpins food ‘liking’ (Robinson et al., 2015). Upon food consumption, dopamine neurons in the ventral tegmental area project to the nucleus accumbens and stimulate dopamine release (Norgren et al., 2006). Research suggests that dopamine release in this circuit positively correlates with self-reported ‘wanting’ (Volkow et al., 2002; Leyton et al., 2002), and excessive dopamine in mice is met by a quicker food approach (Pecina et al., 2003). Findings suggest that increased dopamine is indicative of greater allocation of incentive salience to food, and thus greater motivation to approach (Bindra, 1978).
Moreover, the rewarding properties of food become associated with food cues via a process of Pavlovian conditioning (Bindra, 1978). As such, viewing or smelling food can evoke a strong motivational drive to approach and consume (Weingarten, 1985). Although, the drive is moderated by the internal physiological state (Toates, 1986). Thus, dopamine underpins food ‘wanting’ in eating behaviour.
Moreover, research suggests that reduced dopamine may cause AN. In AN patients there is reduced homovanillic acid (HVA), a dopamine metabolite, which appears to normalise upon recovery (Kaye et al., 1984). This suggests that dopamine dysregulation may have caused the onset of AN. Although, more recent research suggests that reduced dopamine may persist into recovery (Kaye et al., 1999). Kaye et al. (1999) assessed HVA in 6 women recovered from restrictive-type AN, 19 women recovered from BN, 13 women recovered from bulimic-type AN, and 18 healthy females. Results revealed significantly reduced HVA in women recovered from restrictive-type AN compared to all other participants. Thus, reduced dopamine may be a trait factor in restrictive-type AN (Kaye et al., 2009), which may explain the high rate of AN relapse (Berends et al., 2018). However, 4 women recovered from AN fell below a 90% average body weight, indicating that they were not fully ‘recovered’ (Garber et al., 2016), and were in a physiologically deprived state (Bargiacchi et al., 2019). As HVA is negatively related to plasma βHBA (Pahl et al., 1985), an index of malnutrition, the reduction in HVA may be the consequence of restriction, and not causally related to AN. As such, reduced HVA in active and recovered AN may not represent trait-related dopamine dysregulation, but rather a consequence of dietary restraint.
Nevertheless, research using positron emission tomography (PET) with [11C]raclopride confirms reduced dopamine in AN. Compared to HVA, the method accurately examines endogenous dopamine (Drevets et al., 2001), whereby differences in [11C]raclopride binding potential are attributed to either D2/D3 receptor affinity/ density, or concentration of dopamine within the synapse (Frank et al., 2005). Frank et al. (2005) used PET with [11C]raclopride to examine dopamine dysregulation in 10 women recovered from restrictive-type AN and 12 healthy controls. Findings revealed significantly higher [11C]raclopride binding potential in the ventral striatum in women recovered from AN, compared to controls. Specifically, as self-reported harm avoidance increased, dorsal caudate [11C]raclopride binding increased. This posits that AN is characterised by decreased dopamine or increased affinity/ density of D2/D3 receptors. Likewise, dopamine dysregulation is met by the established AN phenotype of harm avoidance (Kaye et al., 2004). Whilst these results indicate that dopamine dysregulation may be a trait-related factor producing AN, causality cannot be established. Rather, dopamine dysregulation may have occurred as a result of AN (Johnson & Bruno, 1990). To establish causality, research should employ a longitudinal design to examine whether reduced dopamine precedes AN onset. Overall, dopamine dysregulation is likely involved in AN, but it is unclear whether it causes AN.
However, PET [11C]raclopride studies do not consistently report dopamine dysregulation. Broft et al. (2015) used PET [11C]raclopride to examine dopamine D2 receptor availability in 25 individuals with AN and 25 healthy controls. All patients with AN were assessed during low weight and upon weight restoration to control for the effects of nutrition upon [11C]raclopride binding. Findings revealed no significant differences in [11C]raclopride D2 receptor binding potential between controls and AN patients at either weight. This indicates that AN cannot be attributed to dopamine D2 dysregulation. Whilst this partially contradicts findings from Frank et al. (2005), dopamine D3 dysregulation may still play a causal role in AN. This is likely given that dopamine D3 receptors adapt to food-restriction in animals (Carr et al., 2003; Carr et al., 2009), and D3 receptor availability increases after AN recovery (Frank et al., 2005), indicative of dopamine D3 dysregulation causing AN. Therefore, dopamine dysregulation in AN may be specific to D3 receptors.
Dopamine dysregulation is further complicated by reports of increased dopamine in food-restricted animals and individuals with AN. Multiple animal studies suggest that food-restriction, a core feature of AN (APA, 2013), results in elevated dopamine in response to rewards, including palatable food (Avena et al., 2008; Carr, 2002). Whilst animal research cannot capture the complexity of human experience, it provides insight into the biological mechanism underpinning dietary restraint in AN. Specifically, animal research suggests that dopamine sensitization is associated with food-restriction (Carr, 2002). This conclusion is consistent with human research. For instance, Cowdrey et al. (2011) assessed neural response, via functional magnetic resonance imaging (fMRI), to food stimuli in 15 women recovered from AN, and 16 healthy women. Findings revealed that individuals recovered from AN displayed increased activity in the ventral striatum for palatable taste, and increased activity in the occipital cortex and prefrontal cortex when viewing palatable food. These findings corroborate with reports of greater dopamine activity in reward regions for patients with active AN (Frank et al., 2012). Notably, women recovered from AN also displayed increased insula and putamen activity in response to the taste of non-palatable food (Cowdrey et al., 2011), and increased anterior cingulate cortex activity when viewing non-palatable food. Whilst fMRI findings do not directly examine dopamine, activity in regions of the mesolimbic circuit is indicative of dopamine transmission (Norgren et al., 2006). Thus, research suggest that individuals with AN have greater ‘wanting’ of both palatable and non-palatable food. This is indicative of greater attribution of incentive salience to food, consistent with sensitization theory (Robinson & Berridge, 1993). Cowdrey et al.’s (2011) findings also confirm prior research of greater cognitive and emotional processing of food in AN (Uher et al., 2004), indicative of greater top-down control in processing food. As such, it is probable that excessive top-down activity, involved in dietary restraint, prevents exposure to food cues that provoke abnormally high ‘wanting’. Based on this evidence, dopamine sensitization may underpin restrictive symptoms of AN.
To conclude, evidence suggests that both dopamine sensitization and reduced dopamine are associated with AN. Whilst the exact reason for the inconsistency in dopamine dysregulation is unknown, it may be attributable to dopamine levels changing as AN progresses. For instance, reduced dopamine may initially cause less food ‘wanting’ which promotes AN development. This is consistent with reports of decreased dopamine (Kaye et al., 1984) and decreased food ‘wanting’ in active AN (Veenstra & de Jong, 2011). For example, an approach-based task for high-fat and low-fat foods revealed that patients with active AN had less food ‘wanting’ overall than healthy controls (Veenstra & de Jong, 2011). As such, those with active AN are less motivated to eat. However, as the food restriction increases in severity the dopamine system becomes sensitized to promote food consumption and facilitate survival (Monteleone et al., 2018). This can explain why presentation of palatable and non-palatable food is met by greater activity in reward circuitry for those with AN compared to healthy controls (Cowdrey et al., 2011). Thus, dopamine sensitization is the compensatory mechanism for food restriction and will only normalise with long-term recovery (Frank et al., 2016). As dopamine becomes sensitized greater cognitive control is employed to prevent exposure to food/ food cues that evoke excessive food ‘wanting’. For instance, AN patients have greater activation of top-down cognitive regions, including the dorsolateral prefrontal cortex, when viewing images of food (Brooks et al., 2011). This illustrates the conflict between physiological motivation to eat and cognitive desire to restrict (Frank et al., 2018). Therefore, dopamine dysregulation is likely dependent on the stage of AN, with reduced dopamine underpinning AN onset, and dopamine sensitization a consequence of restriction.
Conversely, BN is characterised by a different pattern of dopamine dysregulation. Specifically, binge-eating behaviour is associated with persistent, increased release of dopamine during food consumption (Avena et al., 2008) compared to typical eating behaviour which displays a gradual decline in dopamine (Rada et al., 2005). Avena et al. (2008) induced binge-eating in rats by implementing an intermittent food schedule characterised by 12 hours of food deprivation followed by 12 hours of free access to 10% sucrose solution and rodent chow. Results revealed that the binge-eating behaviour caused repeated release of dopamine from the nucleus accumbens. These results are congruous with findings by Rada et al. (2005) who found that binge-eating in rats increased dopamine in the nucleus accumbens by 130%. These findings are indicative of greater ‘wanting’ of food, which is consistent with fMRI findings of increased activity in reward regions of the brains of BN patients when viewing images of food (Brooks et al., 2011). Not only is greater dopamine released in binge-eating, but there is reduced removal of intrasynaptic dopamine evidenced by lower striatal dopamine transporter activity in individuals with BN (Tauscher et al., 2001). As such, binge-eating is characterised by dopamine sensitization which causes greater food ‘wanting’ in BN. However, increased dopamine may be the result of consuming a high-fat diet (McGuire et al., 2011), rather than a determinant of binge-eating. This argument can be disputed as sham-feeding research on rats has found increased dopamine in the nucleus accumbens even when ‘purging’ occurs via the immediate removal of food through an open gastric fistula (Avena et al., 2006). Thus, increased dopamine is not the result of digesting certain foods, but rather causes binge-eating and increased food ‘wanting’.
However, conflicting research reports decreased dopamine in BN. Numerous studies have reported decreased HVA in individuals with BN compared to healthy controls (Jimerson et al., 1992; Kaye et al., 1990). Whilst this appears to complicate our understanding of dopamine dysregulation in bulimia, the findings have been criticised for several reasons. Firstly, HVA is an indirect and unreliable monoamine measure (Kaye et al., 1999). Secondly, critical analysis of HVA findings revealed that the reduction was state-dependent and relied on binge frequency (Jimerson et al., 1992). Therefore, reduced dopamine is likely a consequence rather than a cause of BN.
Not only is dopamine dysregulation involved in binge-eating, but it may also explain purging behaviour in BN. Whilst there are no studies that have examined dopamine release during purging episodes in humans, clinical reports indicate that purging behaviour is rewarding (Broft et al., 2011). Moreover, BN patients report rewarding effects of purging such as a ‘high’ (Halmi, 2009). As a result, purging is positively reinforced by the increase in positive affect (Peterson et al., 2021). Given that positive affect is associated with dopaminergic projections from the ventral tegmental area to the striatum (Peterson et al., 2021), reinforcement of purging is likely caused by dopamine. Specifically, dopamine in the nucleus accumbens may underpin the immediate positive affect of purging, and dopamine in the dorsal striatum may underpin reward learning which maintains the motivational drive to purge (Zhao et al., 2019). Whilst dopamine likely underpins the ‘want’ to purge, the direction of the dopamine dysregulation is currently unknown.
Notably, transition between EDs is the norm (Eddy et al., 2007), and dopamine dysregulation may explain this. As hypothesized, decreased dopamine may cause the onset of AN. As AN increases in severity dopamine systems may become sensitized to aid survival (Monteleone et al., 2018). Dopamine sensitization causes excessive ‘wanting’ (Avena et al., 2008; Carr, 2002) which is moderated by cognitive control (Brooks et al., 2011). As a result of cognitively-induced starvation in the presence of excessive physiological ‘wanting’, binge-eating is likely to arise (Castellini et al., 2011). Negative affect occurs following binge-eating and is responded to by purging behaviour that provides temporary relief (Lingswiler et al., 1989). This illustrates how individuals with AN may transition to BN as a result of dopamine sensitization, and explains why more than 50% of AN cases transition to BN (Eddy et al., 2007; Bulik et al., 1997). This transition has led Eddy et al. (2008) to propose that AN is the restrictive phase of an ED, rather than a distinct subtype, which commonly progresses to BN. However, a small proportion of BN occurs without previous AN (Eddy et al., 2007) and thus may be due to intrinsic dopamine sensitization. Therefore, as the direction of dopamine dysregulation changes, ED symptoms are altered, and diagnostic categories are crossed.
This essay has established that dysregulation in brain dopamine systems is involved in AN and BN, although there is no current human evidence for causality. Specifically, AN onset is likely caused by decreased dopamine, which becomes sensitized by food restriction. Conversely, BN may result from dopamine sensitization following restrictive-AN or reflect intrinsic dopamine sensitization. Although evidence indicates dopamine dysregulation in both EDs, individual differences must be acknowledged. Given that dopamine transmission and activity in reward regions are moderated by sex (Diekhof et al., 2021), EDs in males may be characterised by a different pattern of dopamine dysregulation. Future research should employ a longitudinal, prospective population design to examine dopamine in males and females prior to the development of AN/BN. If dopamine dysregulation is found to cause AN and BN then the dopamine reward circuit should be a target for ED treatments (Frank et al., 2018).
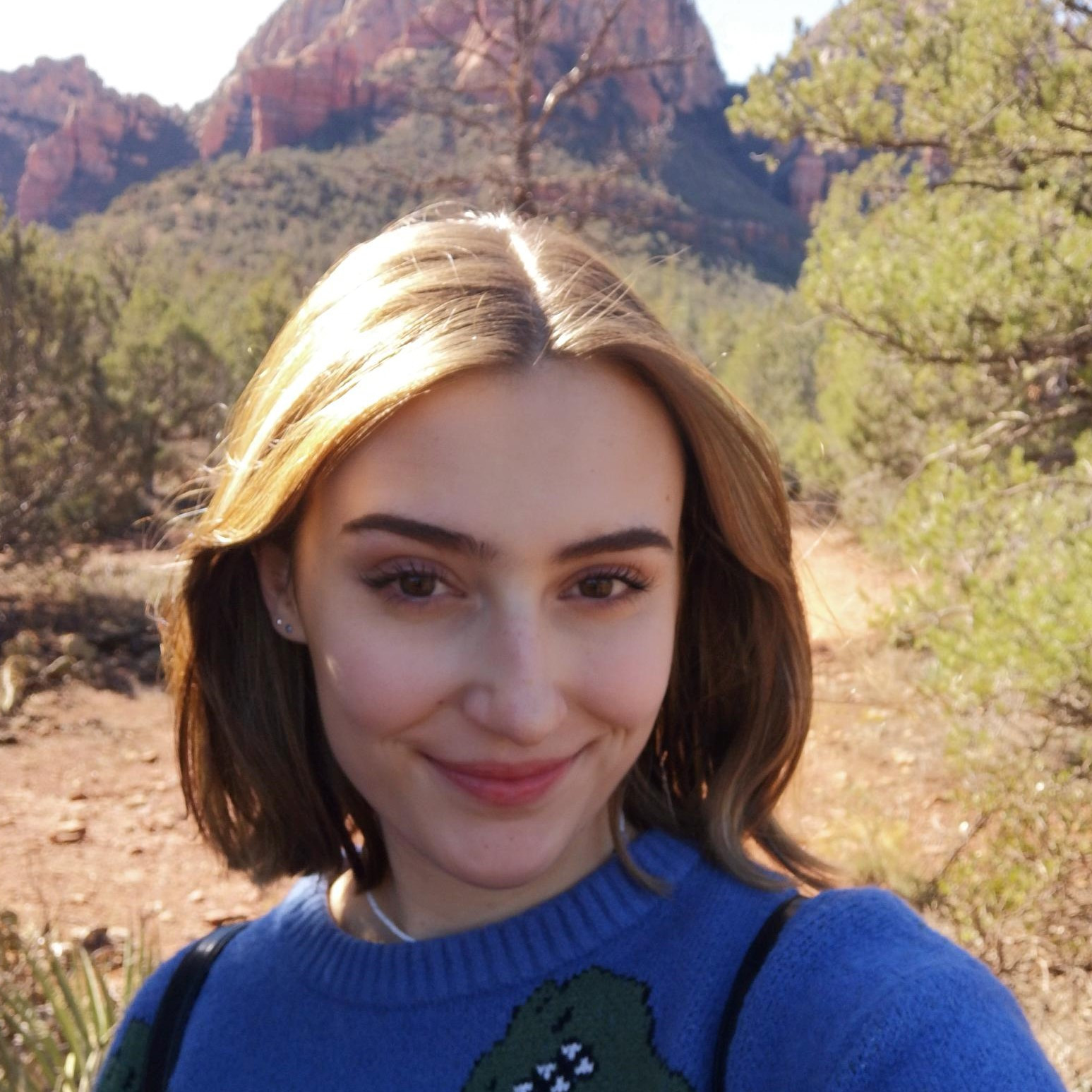